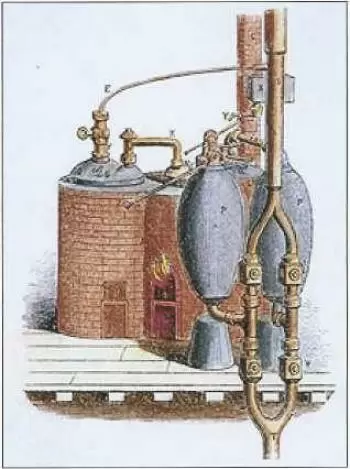
Thermodynamics history is a fundamental stage in physics, chemistry, and general science history. This concept is also related to technological advances such as electricity generation and thermal energy use.
The development of thermodynamics continued and continued with atomic theory. Furthermore, with a creative approach, it mobilized new directions in probability and statistics; see, for example, the thermodynamics timeline.
The foundations of statistical thermodynamics were laid by physicists such as James Clerk Maxwell, Ludwig Boltzmann, Max Planck, Rudolf Clausius, Johannes van der Waals, and the chemical engineer Josiah Willard Gibbs.
What is thermodynamics?
Thermodynamics is the branch of classical physics that studies and describes the thermodynamic transformations induced by heat and work in a system.
These transformations result from physical processes that involve changes in the state variables of temperature and energy.
Timeline of thermodynamics
The history of thermodynamics marks its beginnings in 1824.
In 1824, Sadi Carnot was the first to show that work can be obtained from exchanging heat between two sources at different temperatures. Through Carnot's theorem and Carnot's ideal machine (based on the Carnot cycle), he quantified this work and introduced the concept of thermodynamic efficiency.
In 1848, William Thomson (Lord Kelvin) introduced the concept of effective thermodynamic temperature using the Carnot engine. This idea led him to the second law of thermodynamics.
In 1850 James Prescott Joule proved the equality of the two forms of energy (then it was believed that the liquid caloric still existed).
At that point, the problem arose that, if it were possible to obtain the total heat of the work, it would not have been possible to get the inverse. Rudolf Clausius came to the same conclusion in 1855 when he presented his inequality for recognizing reversible processes from irreversible ones and the state of the entropy function.
In 1876 Willard Gibbs showed how a process could be shown graphically and how studying energy, entropy, volume, temperature, and pressure could foresee the eventual spontaneity of the process under consideration.
The case of thermodynamics is emblematic in the history and in 'the epistemology of science: it is one of those cases in which the practice has pioneered the theory itself: the first was designed for the steam engine, then its theoretical operation was systematized through its basic principles.
In 1967 the first steam engines appeared. At this time, the study of thermodynamics had a practical application in the industry that later sparked the Industrial Revolution.
What is the origin of thermodynamics?
Ancient civilizations viewed heat as something related to fire.
The ancient Egyptians considered heat related to mythological origins. Empedocles proposed a four-element theory, in which substances derive from the soil, water, air, and fire. The Greek philosopher Heraclitus argued that fire, soil, and water were the three main elements.
In the modern period, heat was thought to be a measure of an invisible fluid known as caloric. The bodies could contain a certain amount of this fluid, which led to the term heat capacity.
European scientists Cornelius Drebbel, Robert Fludd, Galileo Galilei, and Santorio Santorio in the 16th and 17th centuries were able to measure the relative "coldness" or "hotness" of the air using a rudimentary air thermometer.
In the 18th and 19th centuries, scientists abandoned the idea of a physical caloric theory and instead understood heat as a show of the internal energy of a system. Today heat is the transfer of disordered thermal energy.
The foundations of atomic theory
Atomic theory is a physical theory that assumes that everything in the world consists of the smallest particles: atoms, held together by nuclear and electrical forces. In the 20th century, it was shown in practice that an atom could be broken down into even smaller subatomic particles.
Atomism is central to the current relationship between thermodynamics and statistical mechanics. Ancient thinkers like Leucippus and Democritus, and later the Epicureans, laid the groundwork for later atomic theory.
Until experimental proof of atoms was provided later in the 20th century, atomic theory and atomic models were driven mainly by philosophical considerations and scientific intuition.
The concept of emptiness
The 5th century BC Greek philosopher Parmenides used verbal reasoning to posit that a void, essentially what is now known as a void, could not occur in nature. This view was supported by Aristotle's arguments but was criticized by Leucippus and Hero of Alexandria.
From ancient times to the Middle Ages, various arguments were put forward to prove or disprove the existence of a vacuum. Multiple attempts were made to build a vacuum, but all were unsuccessful.
In 1643, Galileo Galilei believed that nature's abhorrence of emptiness is limited. Pumps operating in mines had already shown that nature would only fill a void with water to a height of 30 feet. Knowing this curious fact, Galileo encouraged his former student Evangelista Torricelli to investigate these supposed limitations.
Kinetic theory of gases
The idea that heat is a form of motion is ancient and was discussed by Francis Bacon in 1620.
During the same years, Daniel Bernoulli shows that this pressure is two-thirds of the average kinetic energy of gases in a unit volume. Bernoulli linked it to Gottfried Leibniz's principle of living force, an early view of the principle of conservation of energy.
John Herapath later formulated a kinetic theory in 1820. However, he used temperature as an impulse instead of force or kinetic energy.
Boltzmann later generalized his distribution to the case of gases in external fields. Boltzmann is perhaps the single most significant contributor to the kinetic theory, as he introduced many of the basic concepts of the theory.
Boltzmann's equation for the distribution function of gas in non-equilibrium states remains the most efficient equation for studying transport phenomena in gases and metals. He showed that its logarithm is proportional to the entropy.
The rise of the first thermal machines
Irish physicist and chemist Robert Boyle built an air pump in 1656, working with English scientist Robert Hooke. Using this pump, Boyle and Hooke noted the pressure-volume correlation: P·V = constant.
At that time, the air was assumed to be a system of stationary particles and was not interpreted as a system of molecules in motion.
The concept of thermal motion emerged two centuries later. Later, after the thermometer's invention, the property's temperature could be quantified. This tool allowed Gay-Lussac to derive his law, leading shortly after that to the ideal gas law.
But, even before the establishment of the ideal gas law, a Boyle associate named Denis Papin built 1679 a bone digester, a closed container with a tight-fitting lid that confines steam until high pressure is generated.
Later designs implemented a steam release valve to prevent the machine from exploding. Observing how the valve moved rhythmically up and down, Papin conceived the idea of a piston and cylinder engine, a reciprocating engine.
Who invented the steam engine?
In 1697, engineer Thomas Savery built the first steam engine based on Papin's designs.
Although these early engines were inefficient, they attracted the attention of the leading scientists of the day. One such scientist was Sadi Carnot, the father of thermodynamics, who in 1824 published Reflections on the Motive Power of Fire, a discourse on heat, power, and engine efficiency.
It marks the beginning of thermodynamics as modern science.
In the years that followed, more variations of steam engines were built, such as the Newcomen Engine and later the Watt Engine.
Over time, these early engines would eventually be used in place of horsepower. Therefore, each engine began to be associated with a certain amount of "horsepower," depending on how many horses it had replaced.
The main problem with these early engines was that they were slow and clumsy, converting less than 2% of the input fossil fuel, usually coal, into valuable work. Hence the need for a new science of engine dynamics was born.