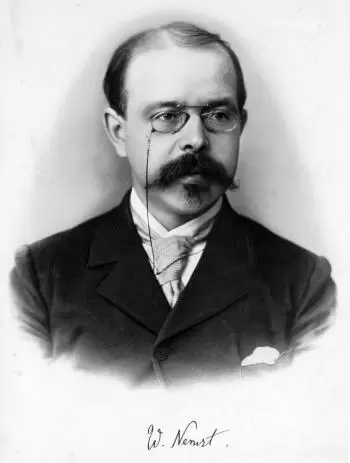
The third law of thermodynamics states that absolute zero cannot be reached in a finite number of steps.
The third law of thermodynamics can also be defined as that when reaching absolute zero - 0 degrees kelvin - any process of a physical system stops. When we are reaching absolute zero, the entropy reaches a minimum and constant value.
The third law of thermodynamics, sometimes called Nernst's theorem or Nernst's Postulate, relates to the entropy and temperature of a physical system.
This principle states that the entropy of a system at absolute zero temperature is a well-defined constant. It is because, at absolute zero temperature, a system is in a ground state, and entropy increases are achieved by degeneracy from this ground state.
Nernst's theorem states that the entropy of a perfect crystal of any element at absolute zero temperature is zero. However, this observation does not consider that real crystals have to be formed at temperatures above zero. Consequently, they will have defects that will not be removed by cooling to absolute zero. As they are not perfect crystals, the information necessary to describe the existing defects will increase the entropy of the crystal.
Theorems and statements of the third law of thermodynamics
The most essential theorems and statements related to the third law of thermodynamics are:
1. Nernst theorem
A chemical reaction between pure crystalline phases that occurs at absolute zero does not produce any change in entropy. In other words: it is impossible to reduce the absolute entropy of a system to its absolute zero value in a finite number of operations.
The third law of thermodynamics allows us to find the absolute value of entropy, which cannot be done within classical thermodynamics.
The German physicist Max Planck used Walther Nernst's heat theorem to define the third law of thermodynamics in terms of entropy and absolute zero.
2. Nernst-Simon statement
The Nernst-Simon statement of the third law of thermodynamics establishes that: “The entropy change resulting from any reversible isothermal transformation of a system process approaches zero as the temperature approaches zero.”
Another version of the law is: “for a condensed system undergoing an isothermal process that is reversible in nature, the associated entropy change approaches zero as the associated temperature approaches zero.”
3. Planck statement
In 1911, Max Planck formulated the third law of thermodynamics as a condition for the disappearance of the entropy of all bodies as the temperature approaches absolute zero.
According to Plank, in any system in equilibrium in which the temperature approaches 0, the entropy tends to be a constant independent of the other thermodynamic variables.
4. Absolute Zero Inaccessibility Theorem
The absolute zero inaccessibility theorem states that:
"A process capable of reducing the temperature of a system to absolute zero would require an infinite number of steps to reach absolute zero.”
5. Callen's fourth postulate
Callen's fourth postulate states that:
The entropy of any system vanishes in the state for which:
What are the consequences of the third law of thermodynamics?
The third law implies the following consequences:
1. It is not possible to reach absolute zero temperatures
The third law of thermodynamics follows that absolute zero of temperature cannot be achieved in any final process associated with a change in entropy. Therefore, it can only be approached.
Therefore, the third law is sometimes formulated as the principle of the impossibility of reaching absolute zero temperature.
Furthermore, absolute zero is the absence of energy. There is no matter if there is no energy since the matter is ultimately energy. The matter is the disturbance of a quantum field that occurs in the presence of energy. Therefore, there will be at least a minimum temperature if there is matter.
2. The behavior of thermodynamic coefficients
Some thermodynamic consequences follow from the third law of thermodynamics: as T → 0, it must also approach zero:
-
the heat capacity at constant pressure and constant volume
-
coefficients of thermal expansion and some similar values.
The validity of the third law of thermodynamics was questioned at one time. However, it was later discovered that all apparent contradictions are associated with metastable states of matter that cannot be considered thermodynamically in equilibrium.