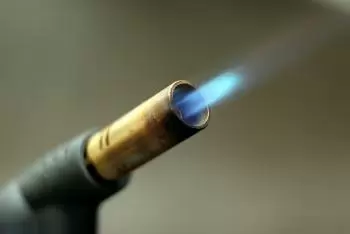
Thermodynamics is the branch of physics that studies the effects of changes in temperature, pressure, and volume of a physical system at a macroscopic level. These systems could be any material, liquid, a set of bodies, etc.
The practical importance lies in the diversity of physical phenomena that it describes. Consequently, knowledge of this diversity has led to enormous technological productivity.
According to the definition of thermodynamics, this science does not study the behavior of each particle but rather the global behavior of all these particles. However, statistical thermodynamics uses molecular properties to predict the behavior of macroscopic compounds.
What does thermodynamics study?
Thermodynamics is the study of interactions between various systems and how they are classified.
A classical thermodynamic system is characterized by its properties, related to each other through equations of state. These can be combined to express internal energy and thermodynamic potentials, helpful in determining equilibrium conditions between systems, spontaneous processes, and the exchange of energy with their environment.
The main elements we have for this study are:
-
The laws of thermodynamics. These laws define how energy can be exchanged between physical systems in the form of heat or work.
-
Entropy. It defines the disorder in which the internal particles that make matter move. In other words, it is the kinetic energy that the particles that make up a body have.
-
Enthalpy is an extensive property which can be derived from the internal energy by adding the product of pressure and volume (intensive properties) as a term. The enthalpy variation between two states at constant pressure is equal to energy transfer.
Laws of thermodynamics
The principles of thermodynamics were enunciated during the 19th century, which regulates thermodynamic transformations, their progress, and limits. They are real axioms based on the experience on which the whole theory is based.
Specifically, three basic principles can be distinguished, plus a "zero" law that defines temperature that is implicit in the other three.
0. The zeroth law of thermodynamics
The zeroth law states that when two interacting systems are in thermal equilibrium, they share some properties, which can be measured by giving them a precise numerical value. Consequently, when two systems are in thermal equilibrium with a third, they are in equilibrium, and the shared property is temperature.
1. First thermodynamic law
The first law states that when two bodies at different temperatures are in contact, heat is transferred to a state of equilibrium. In this new state, the temperatures of the two bodies are equal.
This law is linked with the law of conservation of energy which says that energy is neither created nor destroyed; it just transforms.
2. Second thermodynamic law
Several statements of the second law of thermodynamics are all equivalent, and each formulation emphasizes a particular aspect. First, he states that "it is impossible to make a cyclical machine that has the only result of transferring heat from a cold body to a warm body" (Clausius statement). Therefore, heat transfer always goes from the hot body to the cold body.
On the other hand, it can also be stated that "it is impossible to carry out a transformation whose result is only that of converting the heat taken from a single source into mechanical work."
3. Third thermodynamics law
The third principle is closely related to the latter and, in some cases, is considered a consequence of the last. In this sense, it can be stated that "it is impossible to reach absolute zero with a finite number of transformations" and provides a precise definition of the quantity called entropy.
Additionally, the third law of thermodynamics also states that the entropy for a perfectly crystalline solid at the temperature of 0 kelvin is equal to 0.
Thermodynamic system
A thermodynamic system refers to a limited area used for thermodynamic research and is the research subject. The outer space of the thermodynamic system is called this system's environment.
The system boundary separates the system from its exterior. This limit can be real or imaginary, but the system must be limited to limited space. The system and its environment can transfer matter, work, heat, or other forms of energy at the limit.
There are two kinds of systems:
-
In open systems, the energy can flow to its surrenders.
-
In closed systems, there is no external exchange of heat. In fact, utterly isolated systems are not real, and they are only used to make calculations.
Thermodynamic cycles
A thermodynamic cycle is a circuit of thermodynamic transformations. They are carried out in one or more devices intended to obtain work from two heat sources at different temperatures or, inversely, to produce through the contribution of work the passage of heat from the source from lower temperature to higher temperature.
The main parameter of these cycles is performance. Thermal efficiency is defined as the work obtained divided by the heat expended in the process.
Thermodynamic properties
Thermodynamic properties are the properties that define and intervene in the thermodynamic state of a system. Thermodynamics is characterized by having a state of equilibrium in which pressure, volume, temperature, and composition are present.
These properties can be classified as extensive or intensive. Among these properties, we find internal energy, entropy, enthalpy, heat, temperature, pressure, volume, etc.
Examples in everyday life
Thermodynamics can be applied to various science and engineering topics, such as engines, phase transitions, chemical reactions, transport phenomena, and even black holes.
Here are some examples of some of its applications:
-
In cooking food.
-
In the automotive industry, most engines are heat engines.
-
In materials science to obtain new materials that have well-defined chemical and physical properties.heat engines
-
Industrial uses to transform raw materials into finished products using machinery and energy.
-
In the architectural design, the thermal transfers between the exterior and the interior of the house are considered, especially in bioclimatic architecture and passive solar energy.
-
Generation of electricity in thermal power plants where thermodynamic processes allow heat to be converted into electricity.