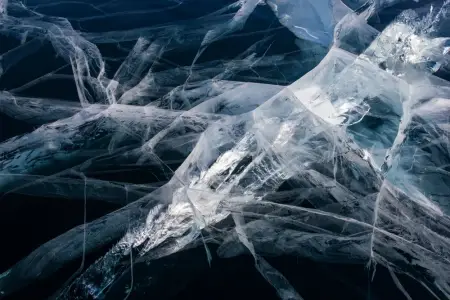
The Third Law of Thermodynamics states that as a substance is cooled to a temperature near absolute zero (-273.15°C or 0 Kelvin), its entropy, which is a measure of the disorder or uncertainty in the system, approaches a constant, finite value.
This law postulates that reaching absolute zero would require an infinite number of steps, making it unattainable in practice. Furthermore, it suggests that all systems would reach a state of maximum order and minimum theoretical disorder at this extreme temperature, which has fundamental implications in fields such as quantum physics and the study of new materials with extraordinary properties at ultra-low temperatures.
Below are some examples that illustrate this principle:
Example 1: Ice crystals
When water is cooled to very low temperatures, close to absolute zero (0 K or -273.15 °C), water molecules lose kinetic energy and organize themselves into a highly ordered crystalline structure, forming ice. Under these conditions, the thermal energy is so low that the molecules barely vibrate and adopt a fixed and regular arrangement.
As the temperature approaches absolute zero, any residual thermal disorder decreases, and the ice crystals reach a state of maximum order, where entropy is at a minimum. Theoretically, at absolute zero, there would be no molecular motion, and the system would be in its lowest possible entropy state.
Example 2: Superconductivity
Superconductivity is a physics phenomenon that occurs in certain materials when they are cooled below a specific critical temperature. At this critical temperature, superconducting materials exhibit a unique property: electrical resistance disappears completely allowing electricity to flow without loss of energy.
The Third Law of Thermodynamics explains the relationship between superconductivity and the reduction of entropy at ultra-low temperatures.
Under normal conditions, when we apply an electric current through a conductor, such as a copper wire, the electrons that carry the electricity face obstacles and collisions with the ions of the material, which generates a resistance to the flow of electrons. This resistance is responsible for the loss of energy in the form of heat and limits the efficiency of electrical devices.
However, in a superconducting material, at very low temperatures close to absolute zero, something amazing happens: electrons form "Cooper pairs." These pairs are composed of two electrons that bind together and move together through the crystal without experiencing any resistance.
Example 3: Liquid helium
Helium is an inert gas at room temperature, but at extremely low temperatures, close to absolute zero, it exhibits fascinating and unique behavior. When cooled sufficiently, below approximately 4.2 K, helium does not solidify like other gases, but becomes a liquid.
As helium cools and becomes a liquid, the helium atoms lose thermal energy, which reduces their agitation and mobility. This decrease in the atoms' kinetic energy results in a significant reduction in entropy. At even lower temperatures, helium exhibits quantum properties, such as superfluidity, where it flows without any friction.
As it approaches absolute zero, the system approaches a state of almost perfect order, where entropy tends to its minimum.
Example 4: Bose-Einstein condensates
At temperatures near absolute zero, some atoms join together in a special state of aggregation called a Bose-Einstein condensation.
In this quantum state, atoms lose their individuality and behave as a single quantum entity. This phenomenon is made possible by the Third Law of Thermodynamics, which states that entropy decreases as extremely low temperatures are reached.
This phenomenon was predicted by Albert Einstein and Indian physicist Satyendra Nath Bose in the 1920s. The idea is based on Bose-Einstein quantum statistics, which describes the behavior of identical and indistinguishable particles, such as photons of light or the atoms that make up certain elements.
Under normal conditions, at higher temperatures, the particles follow a Fermi-Dirac (for fermions) or Maxwell-Boltzmann (for bosons) statistical distribution.
However, when particles are cooled to extremely low temperatures, their collective quantum behavior begins to dominate, and they tend to "collapse" into the lowest possible energy state. At this point, a large number of particles occupy a single quantum state, forming what is known as a Bose-Einstein condensate.
In this quantum state, particles lose their individuality and behave as a collective "superparticle", with macroscopic quantum properties. The entire substance becomes a single quantum entity.
Example 5: Solid helium
At temperatures close to absolute zero, liquid helium can also solidify. In its solid state, helium exhibits unusual behavior, such as superfluidity, where it can flow without resistance through extremely narrow capillaries, defying the classical laws of physics.
Example 6: Dry Ice
Dry ice is carbon dioxide (CO₂) in a solid state at temperatures much lower than the freezing point of water. Unlike water, which freezes at 0°C, CO₂ solidifies directly into dry ice at a temperature of -78.5°C (-109.3°F) at normal atmospheric pressure.
When dry ice is at temperatures close to absolute zero, it behaves similarly to other solids at those extremely low temperatures. The CO₂ molecules that make up dry ice dramatically reduce their kinetic energy, resulting in a highly ordered structure and a significant decrease in entropy. In this state, dry ice would reach its maximum possible thermal ordering at room temperature.
Dry ice is widely used in applications as a refrigerant, in the food industry, in the transportation of heat-sensitive materials and as a special effect in the theatre and entertainment industry.